Chapter 6 – Groundwater and Rivers
Groundwater and Rivers
Learning Objectives
Welcome to Chapter 6. At the end of this chapter you will be able to:
- Define the role streams play in the movement of sediment.
- Identify the reservoirs of precipitation (where it comes from and where it goes)
- Define the water table and explain the difference in the pore spaces above and below the water table.
- Explain how the water table reacts during wet periods versus dry periods.
- Explain how rivers adjust in order to maintain a balanced transport budget between sediment load and water.
- Identify the two main types of river channel and describe how sediment load can determine the type of river channel that will form.
- Explain what happens to stream flow velocity and sediment transport as it enter a larger body of water like a lake or the ocean.
- Compare and contrast a healthy balanced river budget and delta formation with the river budget related to the Mississippi River
- Identify the processes that lead to cave formation in limestone.
- Identify ways in which contaminants can be removed from aquifers that have been polluted.
River Processes – Canyonlands National Park

Southwest of Moab, Utah lies Canyonlands National Park. A “rough” park with few services available, little water (except for the rather muddy rivers), pot-holed roads, and awesome mountain biking, Canyonlands preserves the confluence of the Colorado and the Green Rivers. Many “visitors” to the park never actually enter it, choosing to gaze down on the Colorado from the vantage point at Dead Horse Point State Park. The two rivers of the park are incised a third of a mile (half a kilometer) or more into the red sedimentary rocks of the Colorado Plateau. Those rocks, mostly sandstones (made from sand) and shales (mud rocks, made from pieces smaller than sand), give the distinctive cliff-slope pattern of the canyons—resistant sandstones form cliffs and cap the flat-topped mesas, while softer shales form slopes.
The great Colorado Plateau, flanked by the spreading regions of the Basin and Range to the West, and the Rio Grande Rift to the east, occupies large parts of Utah and Arizona plus some of Colorado and New Mexico, and includes Zion, Bryce, Capitol Reef, Arches, Grand Canyon, Petrified Forest and Mesa Verde National Parks as well as Canyonlands, and many national monuments and other public treasures. The Colorado Plateau is noted for reddish, flat-lying, rocks from the Paleozoic of a few hundred million years ago.

The details are not all known of how the Colorado Plateau avoided extreme deformation for hundreds of millions of years, while most of the rest of the west was being bent, broken, or erupted. The silica-rich continental rocks of the Plateau may be a bit thicker than in its surroundings, so the spreading of Death Valley was unable to tear the Plateau apart and instead jumped across to the east side of the Plateau to continue as the Rio Grande Rift (the valley in which the Rio Grande flows). The spreading even seems to have nibbled away at the Plateau, with a few big pull-apart, Death-Valley-type faults visible in places such as Red Canyon just west of Bryce (see the picture below). However, our concern here is slightly different— the role of streams.

Rain, Rocks, and Rivers
The rivers of the Colorado Plateau are nearly as well known as their parks, for great rafting, incredible scenic views, and deep canyons. The Colorado, the Green, the San Juan, the Fremont, the Virgin and others have taken their place in history. But what are those rivers doing down there in the canyons?
Simply put, a stream or river is a conduit to take excess water, and sediment, from high places to low ones and usually to the ocean. Looking first at the water, rain or snow falls on the ground. Averaged around the world, rainfall (plus snowfall after it melts) is about 3 feet per year. Pennsylvania’s annual rainfall is also right near the global average, as is much of the tree-covered eastern US. Some of this water evaporates directly, but most is used by plants and then is transpired (evaporates) from them. The evaporation from plants and from other surfaces usually is lumped together and called “evapotranspiration.” In a humid temperate climate such as central Pennsylvania, roughly two-thirds of the rainfall is involved in evapotranspiration and returned directly to the sky; in dry climates, a larger fraction of the rainfall—maybe almost all of whatever rain falls—may be returned to the air by evapotranspiration.
Of the water that avoids evapotranspiration, a little actually falls on lakes or streams, and some may fall on the land surface and then flow directly and immediately over the surface into lakes or streams, especially from the surfaces that humans have covered with buildings, roads, and parking lots, thus keeping the rain from soaking in. But most of the rain that avoids evapotranspiration soaks into the ground to form groundwater.
Soils and most rocks include interconnected spaces, either gaps between grains of sand, the cracks in the rock called joints, caves, or other openings. The ground acts a bit like a sponge, with water soaking in and then slowly draining out to the rivers. We will discuss this groundwater flow a bit more when we visit Mammoth Cave, next; for now, simply note that because gravity pulls water down, rocks near the surface usually have some air in the spaces even where conditions are damp, and deeper rocks usually have all their spaces filled with water. The surface separating the rocks with water-filled spaces from those containing some air is called the water table, and where the water table intersects the surface of the Earth, a stream or lake occurs.
Rivers flow even when it isn’t raining because water is slowly draining through the ground from beneath hills to the rivers. The water table rises in elevation during wet times as the “sponge” of the Earth fills up with rain, and the water table falls during dry times as the sponge drains to keep the streams flowing. And, the water table is just below the surface in valleys, actually hits the surface at streams, but you must drill deeper under ridges to penetrate the water table and complete a water well.

As we saw back at the Badlands, weather attacks rocks to produce loose pieces through the processes of weathering. And as we saw at the Gros Ventre slide in the Tetons, processes on hillslopes including soil creep and landslides deliver the loose pieces (which we can call sediment) to rivers. A river is then faced with a balancing act; it must transport both the water and the sediment delivered to it.
You may be able to think of many ways for the river to adjust if more or less water, more or less sediment, or bigger or smaller or “stickier” pieces (those more likely to clump together) of sediment are delivered. For example, if more sediment is delivered to a river than it can remove, then the sediment will pile up, raising the elevation of the bed of the river. This steepens the river—the elevation of the ocean where the river ends has not changed, but the elevation of the river bed away from the ocean is now higher—so the river flows faster and is better able to move the sediment. Deliver more water and less sediment, and the river will wash away all the sediment and have energy left over to carve into the river bed. This cutting downward will make the river less steep—because the river ends at sea level and can’t lower that, lowering the upstream reaches of the river must make the slope to the ocean less steep. A less-steep river will carry less sediment and so quit cutting its bed—the river tends to reach a balance in which it just removes the water and sediment supplied to it. In the process of reaching this balance, rivers also may adjust the width and depth of their channels as well as the steepness.
Rivers are diverse; a white-water rafter on the Youghiogheny River sees a very different setting than greets a river passenger on the Mississippi River going to New Orleans! Of the many river patterns, we often focus on two: meandering and braided (the Mississippi is a meandering river; the “Yough” has some meanders, but not nearly so well developed as the Mississippi). Whether a river meanders or is braided depends on the sediment supplied to the river as well as the water.
A river moves small sediment particles up in the water (suspended load), and larger pieces by rolling or bouncing them along the bed (bed load). If the river receives mostly small particles—what geologists call “fine-grained sediment”—the river typically will form a deep channel. Such a channel is especially efficient at transporting water, and the sediment in the water, because most of the flow is kept away from the river bed and river banks where friction with tree roots and other things slows the water. Very fine sediment—clay—can make fairly deep and steep river banks without collapsing, because the clay particles stick to each other—if you’ve ever worked with clay in art class, you know how well the clay particles stick together, and how much easier it is to make a pot out of clay than out of gravel or sand!
Such deep streams typically curve back and forth, or meander, along their paths. Put a tree’s roots in the way of the stream, and the water flow will curve around the obstacle. In doing so, the stream will race along the outside of the curve and erode it, and a meander bend will grow. (Meandering has been observed to occur without any tree roots in the way, and so is a bit more complex than just curving around obstacles.) Meandering streams usually occur in relatively flat, lowland regions towards the coast.

If a stream receives lots of sand and gravel or even bigger chunks, the large blocks will tend to plug, or dam, a single, deep and narrow channel. The stream then assumes a wide, shallow pattern that is efficient at rolling debris. When sand and gravel get really wet along a river bank, they cannot form steep slopes, as we saw with the mass movements in the Tetons, and the collapse of any steep slopes that start to form along a river bank contributes to having a wide, shallow stream. Within this broad stream bed, gravel (or boulder) bars often form when floods are ending and losing their ability to roll lots of sediment; the water then must flow around these bars. The splitting and rejoining of channels around bars, when viewed from above, looks something like ropes of water that have been braided together, so these are called braided rivers. They are common in upland regions, where steep mountain slopes shed landslides of coarse rocks into the channels.

Again, please recognize that this is a very simple view of stream processes. You can find meandering streams in the flat bottoms of valleys in which beaver dams have trapped mud, for example. Streams with bedrock rather than sediment in their beds often have relatively straight single channels, as do many small streams with sediment beds. The key thing to remember about a stream is that it exists to move sediment as well as water.
A tremendous example occurs a little ways downstream of Canyonlands and upstream of the Grand Canyon. The Glen Canyon Dam was built on the Colorado River in the 1960s. The dam stopped floods coming through Canyonlands from reaching the Grand Canyon—water from floods that had raged through the canyon now is stored in the reservoir and then released gradually. Several things began happening to the river once the dam was completed. The dam trapped the sediment carried by the river, and released clean water, so the reservoir is filling with sediment, and in a few centuries or less will be full. Unique fish species that thrived in the muddy waters of the Grand Canyon suddenly were easy prey for clear-water species that were introduced, so many of the native species are endangered and disappearing now.
With no floods raging down the canyon, high water no longer piled up sand in corners of the canyon to make sand bars. The clean water was able to carry some sand and smaller pieces of sediment, so the existing sand bars below the dam were slowly washed away. The many types of wildlife that depend on sand bars thus were harmed—cottonwood trees that rooted in the sand, birds that lived in the cottonwoods, deer that came down to drink from the river by standing on the sand bars rather than the rocky cliffs. Floods on un-dammed side streams continued to dump large rocks into the Colorado, but the Colorado lacked the high flows to move this material onward, so the rapids at the mouths of side canyons began to steepen.
In the spring of 1996, an artificial flood was released from the dam to attempt to re-balance the system. Indications are that this flood was a partly successful experiment, rolling some of the big rocks out of the rapids at the mouths of the side streams, freeing sand trapped beneath, and forming that sand into bars. But, those bars weren’t very big and didn’t last very long. Additional human-made floods were released in 2004 and 2008, timed to occur when natural floods coming down side streams were delivering additional sediment, to help make bigger sand bars. Additional attempts may be made. Such human-caused floods cost money (lost hydroelectric power when extra water is routed around the hydroelectric plant in the dam to get water into the river in a hurry) and require lots of planning (you need to warn people camping or hiking in the Canyon before you suddenly flood them out!), and they can never get the Canyon back the way it was originally, but the artificial floods do seem to have helped restore some of the sandbars that are so critical for wildlife.
Meanwhile, upstream of the dam, as sediment builds up to fill the reservoir, sediment will also accumulate along the river upstream of the reservoir. There aren’t many people living there, but if there were, their fields and houses would begin to be buried by mud. A river slows down as it enters a reservoir, or any other lake or the ocean, and sediment is dropped from the slowing water. Unless strong waves and currents in the reservoir or ocean take that sediment away, a pile called a delta forms. But, the delta cannot be perfectly flat on top. If it were, then the stream would drop its load when it hit the flat spot and slowed down, and that would raise the flat spot. So, as the delta grows into the lake, the upstream end of the delta must build up so that the river still flows downhill, and that, in turn, will cause sediment to build up for some distance upriver (see the figure below).

The troubles with the dam on the Colorado at Glen Canyon are not unique. Reservoirs immediately start filling with sediment, and so cannot last forever. This filling contributes to deposition of sediment upstream, which may bury houses or fields. The clean water released tends to pick up fine-grained sediment below the dam, but the lack of floods means that the river is no longer able to move coarse-grained sediment. Hence, the river bed is changed, affecting species that lived there. The lack of floods often has huge effects on the plants and animals that live along the river; what had been a region reserved for wildlife adapted to the changing water levels and channels becomes a place for humans to settle in the shadow of the dam.
When two dams were built on the Elwha River, which flows north from Olympic National Park, the dams contributed to blocking salmon runs, to washing away the sand and gravel in which the salmon had spawned downstream of the dam sites, and to turning an annual “flood” of 300,000 salmon into a trickle of barely 3,000 salmon. (A fish hatchery was built instead of fish ladders around the first dam, but the hatchery was quickly abandoned.) Once the spawning bars were washed away below the dam, the river quit delivering sediment to the beaches of the Strait of Juan de Fuca (an arm of the Pacific Ocean); as the beaches washed away, the native peoples were no longer able to carry on their traditional shell-fishing, and engineering was required to protect the nearby harbor of Port Angeles, which was no longer guarded by sediment-fed bars. In an ambitious plan to help the beaches, the river, the salmon and the park, the federal government purchased the dams. One was removed in spring of 2012, with the second slated to follow quickly. The river, beaches, harbor and more will take a while to get back to “normal,” because so much was changed by the dams, but there is much optimism about the recovery. You can read more, and track the progress, at the official website for Olympic National Park.
Controlling Rivers – Delta National Wildlife Refuge

At the tip of the Mississippi Delta lies the Delta National Wildlife Refuge. This is one of several wildlife refuges along the Gulf Coast that, in addition to spawning a great range of resident wildlife, draw migrants from the north. Ducks and geese, herons and cranes, gallinules and rails, the wetland birds of most of a continent stream in through the fall, and then spread north again in the spring. (Yes, technically, a National Wildlife Refuge is not a National Park, but it is a national park, so we’ll cheat a little and use it—it makes a good story. And, it is just down the river from the wonderful bayous of the Barataria Reserve in the Jean Lafitte National Historical Park and Preserve—be sure to stop if you’re in the area!)
Unfortunately, these wetlands are disappearing at an astonishing rate, because of the indirect effects of human activities. Estimates are that every year Louisiana is losing over 100 square kilometers of wetlands (equal to loss of a square with sides more than six miles long). Whether the wetland birds will continue to stream north for generations to come may depend on how humans respond to the challenge.

The Mississippi Delta is a massive pile of mud and sand from the Rockies and Appalachians, transported by the river and dumped into the Gulf of Mexico. The Gulf used to extend far into the heartland of what is now the U.S.; over the last 70 million years, the delta has grown southward from near Cairo, IL (up by St. Louis), until now the former embayment has been turned into a projection from the end of Louisiana into the Gulf. There, the delta is as much as seven miles thick. If you have ever watched mud settle in a bottle of water, or if you have observed how your boot packs mud down if you step in it, then you know that, over time, mud will compact under its own weight or under the weight of anything placed on it.
As the delta grew into the Gulf over the millennia, a natural balance was reached. The compaction that occurred during a year would leave a little space at the top, but the springtime floods would bring new mud to fill the space. Trees and other vegetation would grow up through the new sediment, or re-seed on top, and the system would continue, wildly productive and vibrantly green.
Wrestling with Mud
There is a problem with this system and humans, however. Many people have settled near the river. Plants can grow up through the mud of floods, but people don’t enjoy having their houses slowly fill up with mud. So, humans have built control structures. We built dams upriver, which trap sediment behind them and which hold some floodwaters in check. Because large floods threaten dams and must be let through, we also built levees along the river in its downstream reaches, great walls that hold the river in. We also dredge the river, deepening it to carry the water—and shipping. The great floods that shoot down the river then do not spread over the floodplain and the delta, depositing fertile sediment to fill the space left by compaction of mud, but instead are piped to the Gulf, where the sediment jets off the edge of the delta to settle in mile-deep water.
Way back in 1996, when the very first edition of this textbook was written, it read:
“Today, much of New Orleans, which does lie on the delta, is well below sea level. A tanker in the river between its levees is higher than the playing field of the Superdome. Rainfall, and water seeping from the river, must be pumped out so that the city doesn’t fill with water. If the pumps were to fail, the city would become a lake. The city steadily sinks deeper, and the levees are steadily raised by the Army Corps of Engineers, as instructed by Congress, to keep the river caged. Meanwhile, the wetlands of the delta, unnourished by new sediment, are sinking beneath the Gulf…”
After 2005, we know how terribly accurate the geological understanding of New Orleans really was. Where natural wetlands should have slowed the waves from Hurricane Katrina (which was not a really big storm by the time it got to New Orleans!), the high waters of the storm surge roared unimpeded from the Gulf. Parts of the levees failed. The pumps failed. The city filled with water, as much as 20 feet deep.
The hurricane showed what geologists (and emergency planners!) had long known, and had long told Congresspersons and Senators and students and others—New Orleans was a disaster waiting to happen, not “if” but “when.” With almost 2,000 people killed, hundreds of thousands of people displaced, and damages approaching $100 billion (that is, more than $300 from every single person in the United States), the danger of ignoring solid scientific evidence and hoping for the best is clearer than ever.
But, the city is rebuilt where it was, the sinking will continue, the loss of wetlands will continue unless many things are changed, and the levees will need to be raised. With the likelihood that the strongest storms will get stronger and sea level will rise in the future (we’ll revisit this later in the semester), the scene will be set for an even more horrific disaster at some future date. Many options are available, including restoring wetlands, filling parts of the city with debris or other materials, moving construction to higher parts of the city, moving out entirely, and more; it will be interesting to see how much of this will be done. But primarily, the donations and tax dollars from the rest of the country after the 2005 disaster were used to rebuild the city directly in harm’s way, with the knowledge that the rest of the country will once again foot the bill when disaster strikes.
Another story is being played out in this region as well. The river wants to leave New Orleans. The city has a love-hate relation with the river, fearing the floods but needing the drinking water and the shipping channel. The river can harm the city rapidly by flood, or slowly by leaving.
To understand this tendency of the river to leave New Orleans, note that especially large, muddy, flood-prone rivers normally have natural levees (which are much lower than the human-made ones). When a flood happens, the water spreads out of the main channel onto the flood plain, the flattish region of river-deposited muds next to the main channel. As the water spreads out into the trees or houses of the flood plain, the flow slows, and the water drops some of its muddy load. Just as the water leaves the main channel, the water is carrying the most load, and the slowing is most prominent, so most of the load is deposited right there next to the channel. Hence, the mud layer from a flood is thicker next to the river than farther away, forming a natural levee. Humans have raised these natural levees in many places.

When we discussed reservoirs, we saw that the delta of sediment formed when a river enters a lake must build up as well as out, so that the river still flows downhill into the lake. The same is true for a river entering an ocean. The Mississippi River, with its levees, naturally dumps mud into the Gulf of Mexico, slowly lengthening and raising the river bed. After a while, the river is a bit like a log flume in an amusement park, following a long path to the Gulf; a break in the levee wall would allow a much steeper, shorter, and more exciting downhill trip. The recent history of the Mississippi Delta is that, roughly every 1,500 years, the main outlet of the river has broken through the natural levee, like a log full of park-goers breaking through a curve in the ride, and the river has then followed that new shortcut. But, as mud is deposited along that new shortcut, it lengthens until it is like a long log flume, and then the river breaks through a side again.
During the 1940s and 1950s, the Mississippi started to break out, into a side stream called the Atchafalaya River. To save the shipping channel and the water supply for New Orleans, the Army Corps of Engineers has used the Old River Control Structure and other dams and levees to allow some water to go down the Atchafalaya while keeping a vigorous flow in the main log-flume channel past New Orleans. During a flood in 1973, the Corps very nearly lost the Control Structure, and the river, when a giant whirlpool undercutting the dam came close to causing it to collapse. The task of the Corps is very difficult, taming immense natural forces as the system becomes more and more out of balance.
(An excellent account of this is given in John McPhee’s book The Control of Nature, 1989, Farrar, Straus and Giroux, New York, which may be a little out of date but is still fascinating, and shows that policy-makers and others were warned about the dangers in the area long before the disaster of the 2005 hurricane.)
Mammoth Cave

Deep beneath the rolling Kentucky hills lies Mammoth Cave. With about 390 miles (almost 630 km) of surveyed passageways, this is the longest known cave in the world, more than twice as long as second-place Jewel Cave (which is in a national monument in South Dakota, and has just under 160 miles or 260 km of surveyed passageways). The great size was achieved when a team of explorers showed that the historical part of Mammoth Cave and the even larger neighboring Flint Ridge Cave were actually connected, followed by a lot more surveying. There is every reason to expect that further connected passages remain undiscovered, and estimates extend as high as 1000 miles of total passageways.
The cave was mined for saltpeter (containing nitrates) for use in gunpowder, especially during the war of 1812. The source was bat guano (the polite name for it) deposited over the ages by great flocks of bats. Native Americans used the cave long before European settlement.
Cave Formation
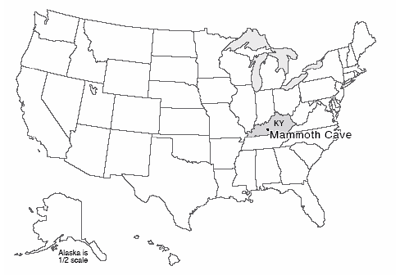
The cave was dissolved in limestone. The limestone was deposited in shallow seas during the Paleozoic (a few hundred million years ago), mostly as shells of sea creatures. The limestone lies beneath sandstone from old beaches. The sandstone is a rather sturdy layer, and provides a “roof” that does not collapse easily, which is important in making Mammoth Cave so big.
As we saw in discussing rock weathering to make muds for the Badlands, rainwater and soil water are weak acids. Chemically, limestone is calcium carbonate, which is especially prone to attack by acid. The usual test for limestone is to drip a little weak hydrochloric acid on a sample; limestone fizzes vigorously as the rock decomposes, freeing carbon dioxide gas, but most other rocks react much more slowly and do not fizz. Where soil waters move through limestone, the rock dissolves and washes away rather rapidly. If the rock has lots and lots of cracks, the water may follow so many different paths and spread out the dissolution so evenly that caves are not formed. But if the limestone has just a few cracks for water to flow through, all of the dissolution will be concentrated in those few places, and cave passageways may form. Then, if the water table is lowered (say, because the nearby river has cut downward through rocks and is draining water from the rocks to its new lower elevation, as Kentucky’s Green River has done near Mammoth Cave), water will drain away and the cave will fill with air.
The beautiful stalaCtites (from the Ceiling), stalaGmites (on the Ground), and other cave formations can then develop. Because processes in soil—worms exhaling, and similar activities—typically release carbon dioxide, groundwater usually contains more carbon dioxide than does rainwater, so groundwater is more effective than rainwater at dissolving limestone. Occasionally, a cave may be so isolated from the surface that dangerous levels of carbon dioxide exist in the cave, but caves usually exchange enough air with the outside world to have near-normal levels of carbon dioxide. When groundwater drips into a cave that has a near-normal carbon-dioxide level, the water loses carbon dioxide to the air. The water then cannot hold all of the dissolved limestone, and deposits some of it to form the beautiful stone features we see.
Sometime, look at a cliff or road cut (but be careful—we don’t want to lose anyone to distracted driving, or being run over along a roadside!). If you look carefully, you should see that almost all cliffs and road cuts have cracks (called joints), and that some of those joints are usually vertical; very often there are two or more sets of vertical joints, perhaps with many joints oriented north-south but many others oriented east-west. Rainwater that is not used by plants will trickle down the “pipe” where the joints intersect. If the rock around the pipe is limestone, the rock will dissolve, leaving space, although that space may fill with mud. Such a hole, whether mud-filled or air-filled, is called a sinkhole.
Sinkholes formed by downgoing waters are very common in the State College, PA area, where Dr. Alley lives. Penn State’s Geosciences Department is housed in the Deike Building, which required extra funding for special strengthening because the building has sinkholes beneath—a building can rest firmly on bedrock, but tends to fall into air-filled or mud-filled holes. Extra funds were similarly expended to strengthen the nearby Mt. Nittany Middle School, the runway extension at the airport, and other construction projects in the area. A newly constructed storm-water catch basin at the airport filled with water during the first rain, and the weight of the water blasted mud out of a buried cave passageway somewhere beneath, suddenly turning Spring Creek red with trout-choking sediment.
Where sinkholes and caves are common, streams often disappear underground into swallow holes, only to re-emerge at springs. Spring Creek is aptly named, and many other similar features occur around central Pennsylvania, around Mammoth Cave, and in other such regions. Corn cobs once were dumped in a sinkhole behind a cannery at Old Fort east of State College, and after a rain would pop out of a spring in Spring Mills, a few miles away. Regions with sinkholes, caves, springs, swallow holes, etc., are referred to as karst, after a region in Slovenia with many such features. Karst features are present across 20% of the Earth’s surface, and roughly 40% of the US population obtains drinking water from karst, according to the National Park Service.
In the past, people often threw trash into sinkholes. Big pieces would sink into the mud or fall into cave passages beneath, “disappearing.” When Dr. Alley was in high school and went to Sloan’s Valley, Kentucky to go wild caving (spelunking), one of the cave entrances was known as the Garbage Pit, which led into the Tetanus Tunnel. A commercial cave near Mammoth Cave was forced to close in the 1940s because of the stench from sewage draining in.
Slowly, we are learning just how stupid it is to dump things in sinkholes. A test run by Penn State’s hydrogeologist, the great Dick Parizek, during the building of the Nittany Mall showed that a little harmless dye dumped in a sinkhole near the mall came out in a nearby trout stream in a day or two. It should be evident that anything else dumped in a sinkhole near the Nittany Mall (or many, many other sinkholes in the region and in other karst regions) would show up very quickly in the water used by people and wildlife.
Dr. Alley lives in a house served by the Lemont Water Company, which is now well-known for fine water. But many years ago, before a reorganization of the water company, the intestinal parasite Giardia showed up in Lemont well water. Giardia causes intense and possibly dangerous gastric discomfort, and is to be avoided. Giardia usually is restricted to surface water; the spaces in most rocks are small enough to filter out the Giardia cysts before they reach a well, or the water takes so long to go from the surface to a well through the small spaces that the cysts die of old age on the way. At the long-ago community meeting to discuss the water contamination, company officials noted that they had installed well filters to remove sticks, leaves, etc., that came out of the wells with the water. In karst country, surface water can become groundwater and return to the surface in hours or days. Whole streams go down and up, and if sticks can go through, microscopic cysts can, too. Clearly, contaminants dumped somewhere today can be poisoning someone tomorrow.
In some other regions, the groundwater-contamination problems are quite different. In sandstones, for example, the water moves slowly, pore-by-pore, through the rocks. In some places, the water can be shown to have first entered the ground during the ice age, more than 20,000 years ago, or even earlier. Contaminants dumped in such rocks may not bother people for a while. But, when the contaminants do start to bother people, clean-up can be very difficult.
Try this experiment. Squirt some soap on a sponge, and squeeze the sponge a few times to distribute the soap well. The sponge is our rock, and the soap is the contaminant. Now, wet the sponge, hold it up, and squeeze it. Foamy, soapy water will come out. Wet the sponge again, squeeze it again, and more soap comes out. Repeat, and repeat, and repeat. You may need ten or more times to remove enough soap that you no longer see it, and sensitive instruments would detect the soap through dozens or even hundreds of additional washings. Now, suppose that we had not soap, but a chemical that causes cancer in humans. If the water in the rocks naturally is hundreds or thousands or more years old, then nature takes a long time to wash out the rocks once, and washing them out ten or one hundred times will take much longer than all of human history.
There are things that can be done about groundwater pollution. You can pump clean water in and dirty water out, or pump steam or hot water in and out (and then try to figure out how to clean the dirty water or steam once you have them on the surface). People are experimenting with installing filters so that polluted water will flow through them, sometimes using large masses of iron filings to react with and break down some organic chemicals in groundwater. Geomicrobiologists are prospecting really dirty sites for “bugs” that “like” to eat pollutants, and then trying to introduce those microorganisms into other polluted sites; other biologists are looking into ways to design pollutant-eating microbes. But, such techniques usually are very expensive and not very effective. Most people who have thought about it agree very strongly that the best way to handle groundwater pollution is to keep the chemicals out of the ground in the first place. A whole lot of money has been spent on clean-up because we did not learn that lesson soon enough—and there are days when it appears that we have not yet learned that lesson.
GeoMations
How Deltas Work
How Deltas Work
Transcript: We’re flying along in our helicopter out over the ocean, and we’re looking towards shore at a beautiful beach we just saw there. And we’re seeing the pretty waves of the ocean underneath us. And we see a great river coming down to the shore, something like this, meandering along the way some of these great rivers do.
Now if we could somehow hang along in our helicopter and wait for a flood, what we’d notice is that sitting next to the river, there’s a bunch of trees that are growing. And when the flood happens, the water would start trucking out of the river into both directions. And as the water came trucking out of the river, it would slow down when it got into the trees.
And as it did so, it would start depositing a layer of mud. And that layer of mud would be thickest very close to the river, and then it would thin on out across the flood plain of the river. And this would be happening on both sides of the river. And so after a while, we would see that the river is contributing to building a natural levee that sits along the river and runs all the way out to the mouth.
Now, if we could keep watching this happen over very long times, over hundreds or thousands of years, we would see that in many places, the ocean is not strong enough to get rid of all the mud that the river delivers. And so the river would start building out into the ocean, and it would just extend its way out there, building a levee. And as it did so– not a very high one– but as it did so, the river itself would lengthen. And so it would be coming out in there, and the water would be flowing down to the sea.
And when this is going on, it has to keep going downhill. So it builds up, as we saw earlier, as well as building out. And so you start getting higher walls that hold the river in, on up here, to allow it to flow downhill and run out like that.
And so as the bed of the river is raised, and it gets higher walls, it’s like being on the log flume at the amusement park. And at some point, there’s a flood, and that wall breaks, and the river takes the short way down to the sea. And then the whole process will start over again. It’ll start building a new wall, and building out that way. And eventually, sometime in the future, this one will become big, and then it will do it over again. And so rivers build deltas. They switch from one place to the other as they build out.
How Dams Work
Transcript: So let’s take a look, a strange look, at a river. This is going along the river towards the sea. And this river happens to have trees that grow up on the riverbank. So you can get an idea how we’re looking at this.
And what we want to do is ask what happens when we build a dam on this river? And we’re concerned about the future of a couple of houses that used to be along the river, one just below the dam right down here, and the other one up above the dam up here, sitting along the riverbank. Well, they build the dam, and the dam fills with water, and it doesn’t quite take out the house up above.
But the river’s carrying mud. It’s carrying sediment. And the sediment starts to deposit out into the lake to fill it with a delta.
Now, rivers have to go downhill. So as the lake is replaced with the mud, if the river were to hit a perfectly flat spot like this, you know what it has to do. It has to build up so that it’s headed downhill. And so as the river builds the delta out into the lake to fill the lake, why, you have to bury the house, and that makes the person who lives there mad.
Now, at the other side, it’s even more interesting. There’s no floods anymore, so the river loses the ability to carry gimongous rocks, which might make the homeowner happy. But the water coming out from the dam is clean. It has no sediment in it. And if there’s sand below the dam, why, the water will start washing that sand away.
And that will do a number of things. Your house, now, rather than sitting there right next to the riverbank– you’ll look out some morning and you’re ready to fall into a giant hole that’s been cut. And you get out there and you fall and then you say, oh, no, and you’re very unhappy.
You are not nearly as unhappy as the salmon that are trying to come upstream, because as you may know, salmon like to get really amorous around sand and gravel bars. And if the river has washed away the sand, then you can’t have fun with your honey and then leave your eggs there to do well. And so you have a big loss in salmon, as well as getting people’s houses unhappy. And so putting a dam on a river makes a big difference.
How New Orleans Doesn’t Work
How New Orleans Doesn’t Work
Transcript: Here’s a great tree. And the great tree is standing up on a bluff looking down on the Mississippi River, which sits down in a valley. And the river has a little natural levee– this, we haven’t gotten to human built ones yet– and it sits down in a valley.
And then, over on the other side, there’s another natural levee, and then you go up another bluff to the top. And the river itself sits down in its river valley like this. And it is flowing towards you, which is what the head of this arrow shows.
And the river is sitting on a few miles thickness of mud that have accumulated over the years as the delta has built out into the Gulf of Mexico. And whenever you get a few miles of mud, everything is sinking under its own weight. And that, in turn, means that the surface is sinking, as well.
Now, nature has a way of handling this surface sinking problem, which is that during a big flood, the water spreads out over the flood plain. And mud falls out of the water, and the mud makes a new layer. And so as the surface sinks, more mud is added. And so there’s no net change in the elevation. And that’s just fine, except for one little problem.
Over here you have a city. And you’ve built this big city. And you really don’t want that flood coming into your city. So you just call up the Corps of Engineers and you say, make a big wall, and make sure that that flood is not going to get into my city.
Well that’s just fine, except that doesn’t stop the sinking, because the thing is going down. And so if you come back later, what you’ll find is that the surface has moved down to a place like this, and your city has moved down to a place like this. I’m using a darker line so you can see where it’s gone to from where it was.
And so you tell them to make the wall bigger. But meanwhile, the city is sinking even deeper. And you’ve gotten up somewhere way down here, now.
And things are getting really nasty, because at this point, there’s a really big storm. And it manages to get just over the top, and it fills your city with water. And then you’re underwater and you’re very, very unhappy. And this happened to New Orleans. And the sinking is not stoppable, so if it’s rebuilt, it is likely that it will happen again.
INCISED MEANDERS AND GEOLOGIC HISTORY
Canyonlands poses a special puzzle. The rivers in Canyonlands meander, making big, sweeping curves through the red rocks. In the Canyonlands chapter, you read that meandering streams occur in flat, lowland regions lacking supply of big rocks to the stream. This is decidedly not the situation in Canyonlands. The streams are steep, and in places (although not just where the picture below was taken) are laden with rapids, challenging for whitewater rafters. Landslides and rockfalls from the canyon walls deliver large blocks to the streams.
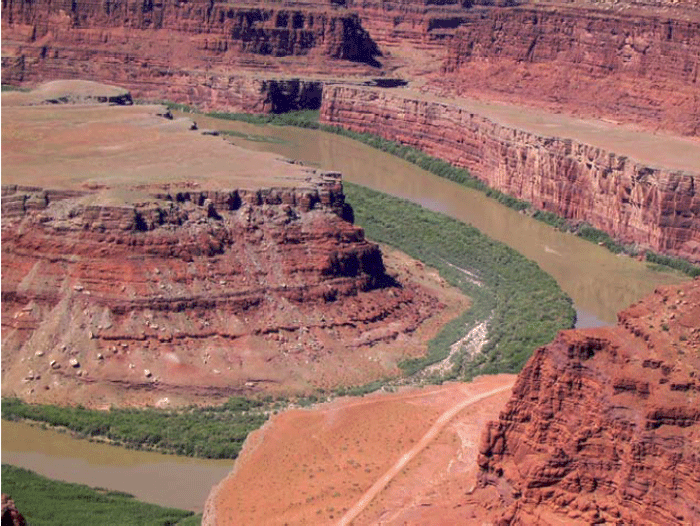
The likely story is that the streams once flowed across nearly flat lowlands. Then, uplift of the rocks began, giving the streams a steeper slope to the sea and so speeding their flow and causing them to erode. But, the uplift was gradual enough that the streams held their old courses. The streams cut downward without a change in pattern, which is called incision. Canyonlands contains clear examples of incised meanders. Similar features are preserved throughout the Colorado Plateau, documenting widespread uplift of the Plateau.
Much of geology involves study such as this; the history of a region produced the modern features. One can often learn much about that history by understanding the modern features and how they were formed. Clearly, if we did not know what conditions produce meandering streams today, we would not know what conditions likely occurred in the past when the meandering streams developed. The geological saying that captures this idea is “The present is the key to the past.”
SINKING MUD
Down at the Mississippi Delta, we saw that the mud under New Orleans is compacting under its own weight, contributing to sinking of the city. A couple of other things also contribute to the sinking.
As the delta grows, the weight of the mud pushes down the rock beneath, with soft, hot rock much farther below flowing away laterally, like water under your behind if you sit on a water bed. The sinking is not instantaneous. And, just as the strength of the water-bed cover spreads the dimple around your behind when you sit down, a fairly large region around the delta is pushed down by the weight of the delta. So add some mud anywhere near New Orleans, and you get a little sinking in New Orleans for a while.
Perhaps more importantly, as we saw with mass movements at the Grand Tetons (and as you can see in the picture of Canyonlands on the previous page, in the cliff on the inside of the meander), rocks tend to fall off steep slopes. Sometimes a single rock falls, or a thin layer of rocks slides. Other times, large thicknesses of material move. The Mississippi Delta is a giant pile of mud towering above the deep waters of the Gulf of Mexico, and that “cliff” is subject to downward motion of its materials. Some of this occurs along faults that are something like pull-apart Death-Valley-type faults—the fault intersects the Earth’s surface well inland, sloping down toward the Gulf, and the mud on top slides down and toward the Gulf.
After Hurricane Katrina devastated New Orleans, geologists renewed their efforts to understand what is going on geologically—such understanding should help in planning how to slow down the next disaster. An argument has erupted about the relative importance of the various reasons for sinking in and near New Orleans, with faulting probably more important (and mud compaction less important) than previously believed, and with sinking of the rock beneath minor but not zero. All are almost certainly contributing, but with a little work to figure out just how much to blame on each one. Naturally, all of them contributed to lowering of the surface, and sediment deposited from the river’s floods filled that space—the river doesn’t really care why the surface dropped, and deposits sediment in low places formed by any process.
Key Takeaways for Groundwater and Rivers
Water, Rivers, Floods, and Caves: Canyonlands, Delta and Mammoth Cave
- Most rain evaporates, most of remainder soaks in.
- Soil and shallow rock usually have air as well as water in spaces; deeper, below water table, spaces all water-filled.
- Water table looks like a smoothed version of the ground surface, and intersects surface at streams.
- Ground is like a sponge that fills during rains, drains to keep streams running between rains.
- Water table rises during wet times, sinks during dry times.
Rivers Move Rocks
- Rivers receive water, and rocks by mass movement.
- If more rocks arrive than water can move, rocks pile up, steepening stream so it can move more rocks.
- Sticky, small clay particles favor a single, deep meandering channel that moves suspended load up in channel.
- Lots of sand, gravel or larger rocks that don’t stick together favor many shallow braided channels.
Dams Make a Big Difference
- Sediment builds deltas to fill reservoirs formed by damming rivers.
- A delta builds out but also up, “backing up” sediment to bury fields and houses for some distance upstream.
- Regions downstream of dams no longer get floods.
- Without floods, big rocks are no longer moved by rivers.
- Clean water released by dams picks up sand, removing sand bars and affecting river ecosystems.
Ignoring rivers can be dangerous
- A delta is a big pile of sediment, which compacts under its own weight.
- The Mississippi delta is miles thick, compacts a lot.
- The natural sinking is balanced by new mud from the annual flood.
- Wetlands below New Orleans have been lost as levees and dredging for shipping kept flood mud from balancing sinking.
- A low city by a high river and sea with no wetlands to slow storm surges brought huge hurricane disaster.
- Scientists, disaster planners, many journalists and others repeatedly warned that this would happen…
- The sinking continues; rebuilding without major changes will cause the next disaster to be even worse.
Caves are Cool
- Some rocks (esp. limestone) dissolve easily; get sinkholes from surface, caves beneath, springs, etc.
- If cave dries, loss of soil CO2 to cave deposits dissolved limestone as cave formations.
- Water goes through caves quickly; pollution discharged today may harm someone tomorrow.
- For other rocks, water moves much more slowly; pollution may not “get” anyone for a while, but once it does, clean-up is very hard and slow.